Your dorm room and Duke washing machines aren’t the only places mold likes to hang out. For a while now, astronauts at the International Space Station (ISS) have also been dealing with a mold problem. Astronauts need to regularly maintain a “clean” environment at the ISS, as water and air are constantly recycled and a biological infestation can pose severe risks to the health of personnel and the completion of their missions. While it is expected that microbes — carried on by humans — are present on the space station, the distinct gravity and radiation conditions have a large impact on microbe composition and survival rate. The first step to assessing the risks coming from these microorganisms is determining what exactly they are: pathogenic bacteria like Salmonella and Clostridium botulinum or “good” fungi such as the penicillin-producing Ascomycota?
There are many ways of determining the species of an organism. When dealing with organisms invisible to the naked eye, researchers must rely on methods to read genomic sequences and differentiate species by their individual genetic codes. This tactic is precisely what scientists employed at the ISS. Instead of bringing microorganism samples back to sequence on Earth (at which point the mold infestation could have already been lethal and varying conditions on Earth could have altered the samples), NASA astronauts set up a small lab right in the space station.
Ten years ago, this project would have been impossible. Imagine carrying hundreds of thousands of dollars worth of massive DNA sequencing equipment to space, only to find out that it was damaged by vibrations during take-off. However, due to rapid biotechnology advancements, NASA was able to complete its first DNA sequencing in space in 2016.
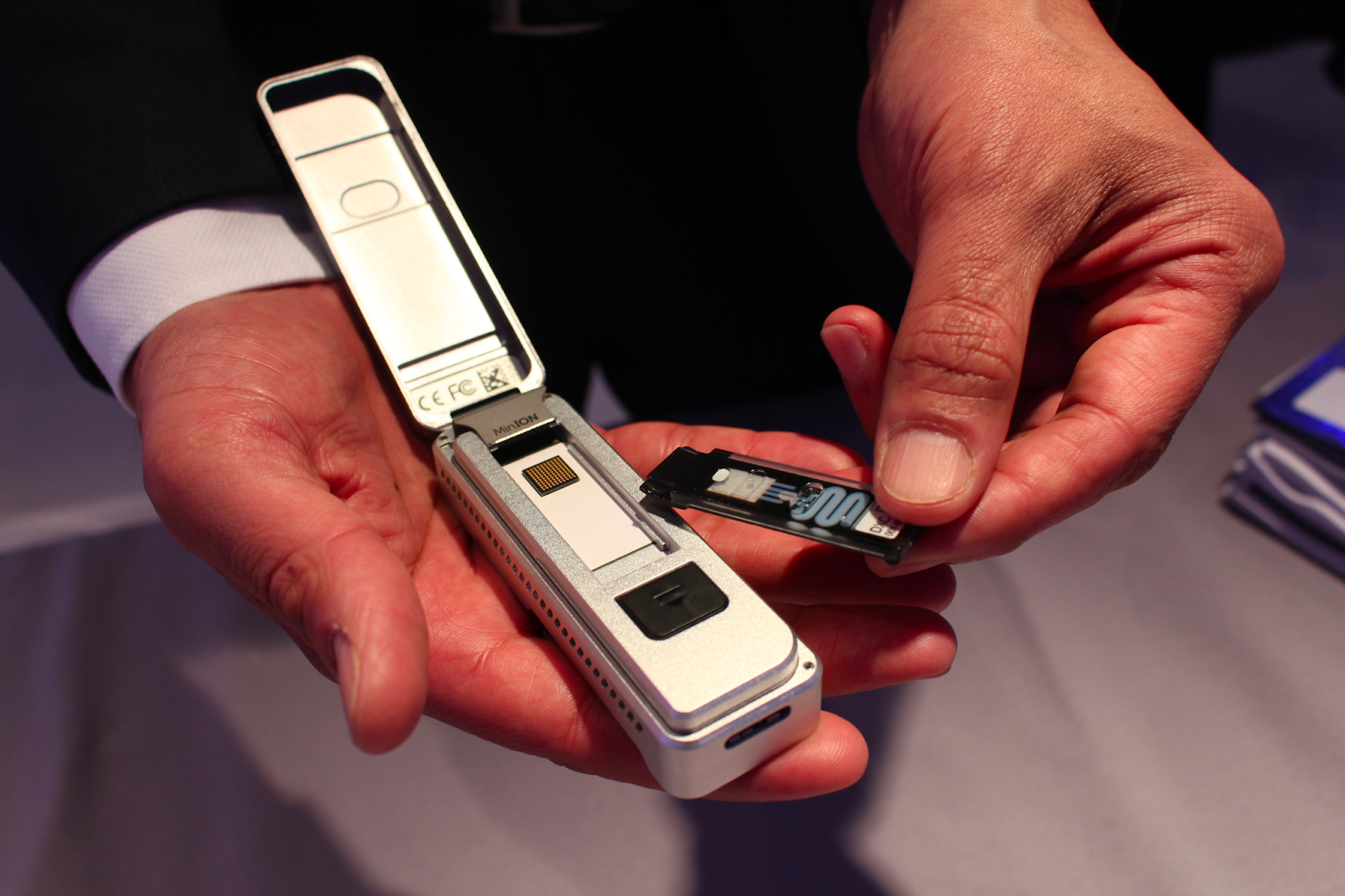
Above: Oxford Nanopore MinION. The device can be connected to a laptop and perform sequencing in a limited-resource environment, such as the ISS or an outdoor research expedition. Courtesy of Oxford Nanopore.
The astronauts relied on a small nucleic acid sequencer called MinION. Developed by Oxford Nanopore Technologies (ONT), MinION is only slightly larger than a flash drive. For reference, older sequencers were as large as a small household refrigerator. MinION is part of a group of sequencing methods called Next Generation Sequencing (NGS), which works with huge amounts of genetic material and offers much faster and more cost-effective results. ONT’s MinION employs a novel method of reading nucleic acid fragments. First, genetic material is pipetted into a flow cell where the sequencing reaction takes place. There, small protein channels called nanopores read the DNA fragments. This method relies on the disruption of an ionic current that passes through the flow cell. As each nucleotide fragment passes through a nanopore, it disrupts the ionic current in its own characteristic way. By measuring the ionic current disruptions, MinION can decode long nucleotide sequences in real-time.

Above: Used Oxford Nanopore flow cell. The nanopores are in the lower center, under “SpotOn.” The pores are organized in columns and rows, forming grid-like structures. The flow cells can be reused up to five times if properly washed, per the producer. Courtesy of Vinicius Fu.
NASA’s successful interstellar DNA sequencing offers a glimpse into the growing automation and optimization of time-consuming processes in biotechnology. In the 1990s, the United States started an international research project called the Human Genome Project to determine the entire human genomic sequence. It took nearly 13 years to sequence about 92 percent of the human genome. With NGS, you can fit multiple human genomes in a single flow cell and sequence them in a matter of hours. Although the equipment still carries a hefty price tag (ranging from hundreds of thousands to millions of dollars), the output of data has also massively increased, resulting in a lower price per base sequenced. Due to high costs, many labs rely on the services of specialized research centers that can afford such machinery. One such institution is the Sequencing and Genomic Technologies (SGT) of the Duke Center for Genomic and Computational Biology. Located at the former Liggett & Myers Tobacco factory in downtown Durham, the SGT receives genetic samples from Duke labs and external institutions and performs various kinds of sequencing — methylation, exome, mi RNA, total RNA, single cell, etc. Each of these techniques requires genetic sequencing machines and reagents that are costly and extremely precise, which is why labs may want to contract out the services to the SGT.
.jpg)
“It does take specialization and training to do [sequencing] and the actual sequencing equipment can be really expensive,” said Dr. Devi Lenz, Associate Director of the SGT. “Another reason is time. If you’re working on multiple projects and you need to get [sequencing] done, you can just outsource to us.”
Above: NovaSeq 6000 flow cells separated in order of increasing genetic material sequencing capability, from lowest (left) to highest (right). Courtesy of Vinicius Fu.
Beyond ONT, the SGT also works with other types of NGS equipment, such as Illumina and Pac Bio. Each technology varies in error rate, cost, and efficiency depending on the type of sequencing desired. For instance, ONT is great for reading long strands of nucleic acids; however, it presents a comparatively higher error rate. Alternatively, Illumina is ideal for short sequences.
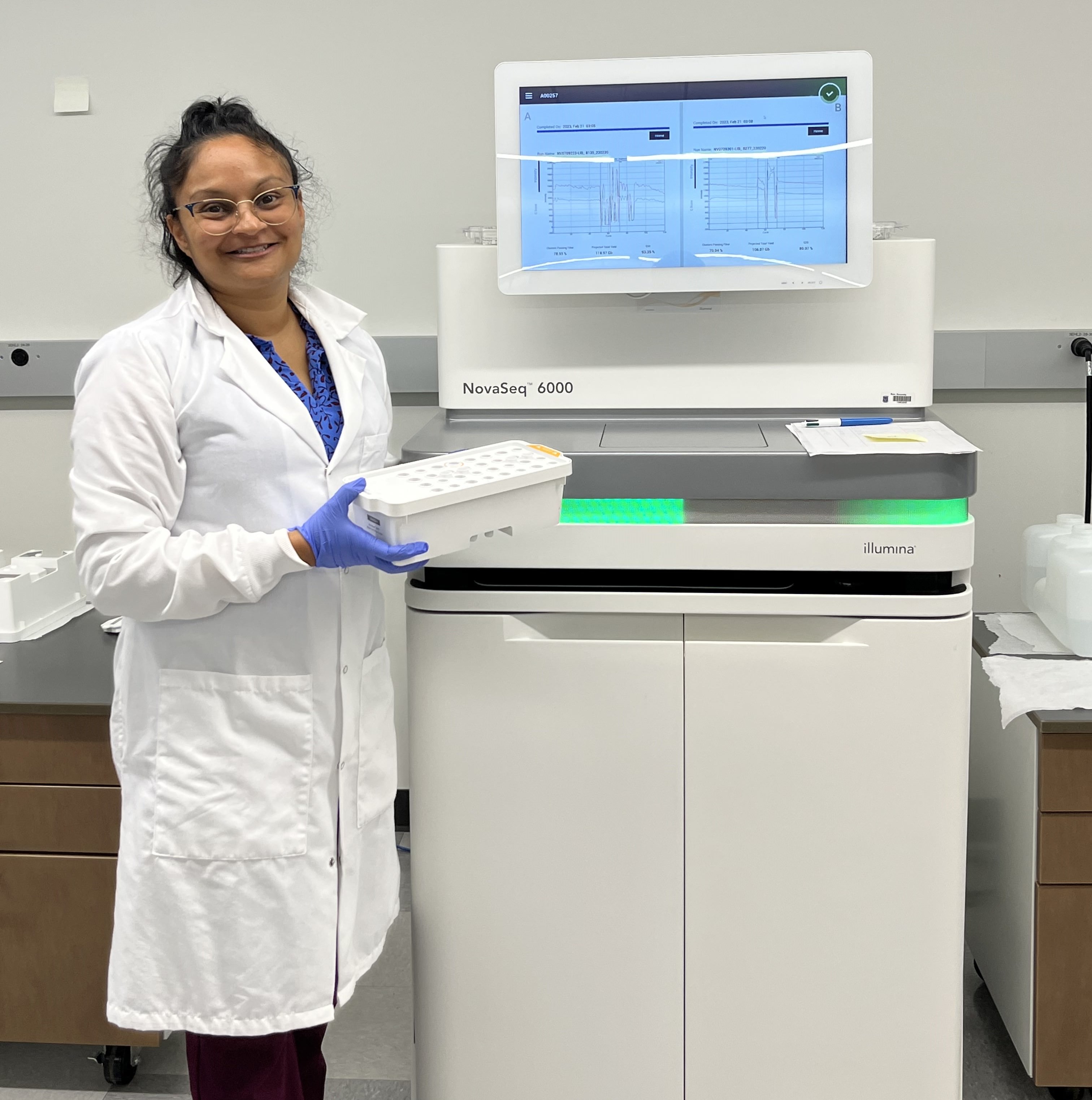
Right: Dr. Devi Swain Lenz, Associate Director of the SGT, stands beside a NovaSeq 6000 sequencer. Many newer high throughput sequencer models are smaller in size. Courtesy of Vinicius Fu.

Left: Illumina NextSeq 1000, a benchtop model with a much more modern visual display. Courtesy of Vinicius Fu.
Since the SGT is not as space-constrained as the ISS and is more focused on high throughput, the facility uses massive apparatuses to optimize workflow. Similar to MinION, ONT has also developed GridION, which can work with five flow cells at a time. The process of preparing the genome that occurs before sequencing is also automatized using a Biomek i7, a sort of “mechanical hand.” Instead of pipetting thousands of samples and amplifying them in a thermocycler, this robot does the repetitive job following the setup determined by lab needs.
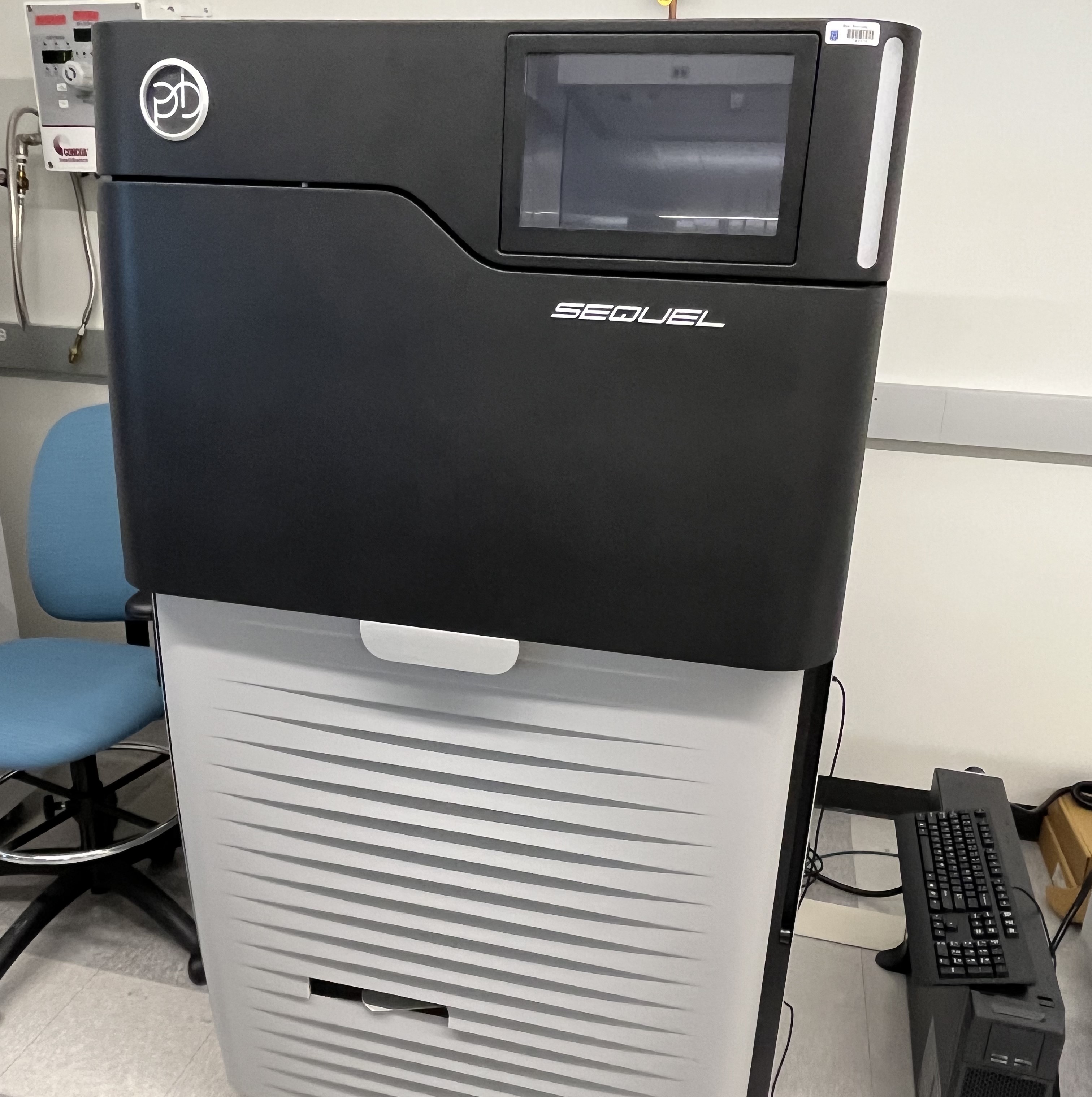
Above: PacBio Sequel sequencer, currently being replaced by a newer model. Courtesy of Vinicius Fu.
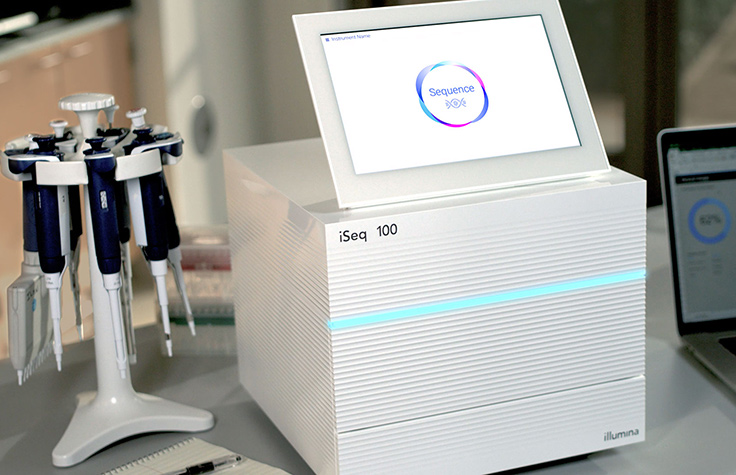
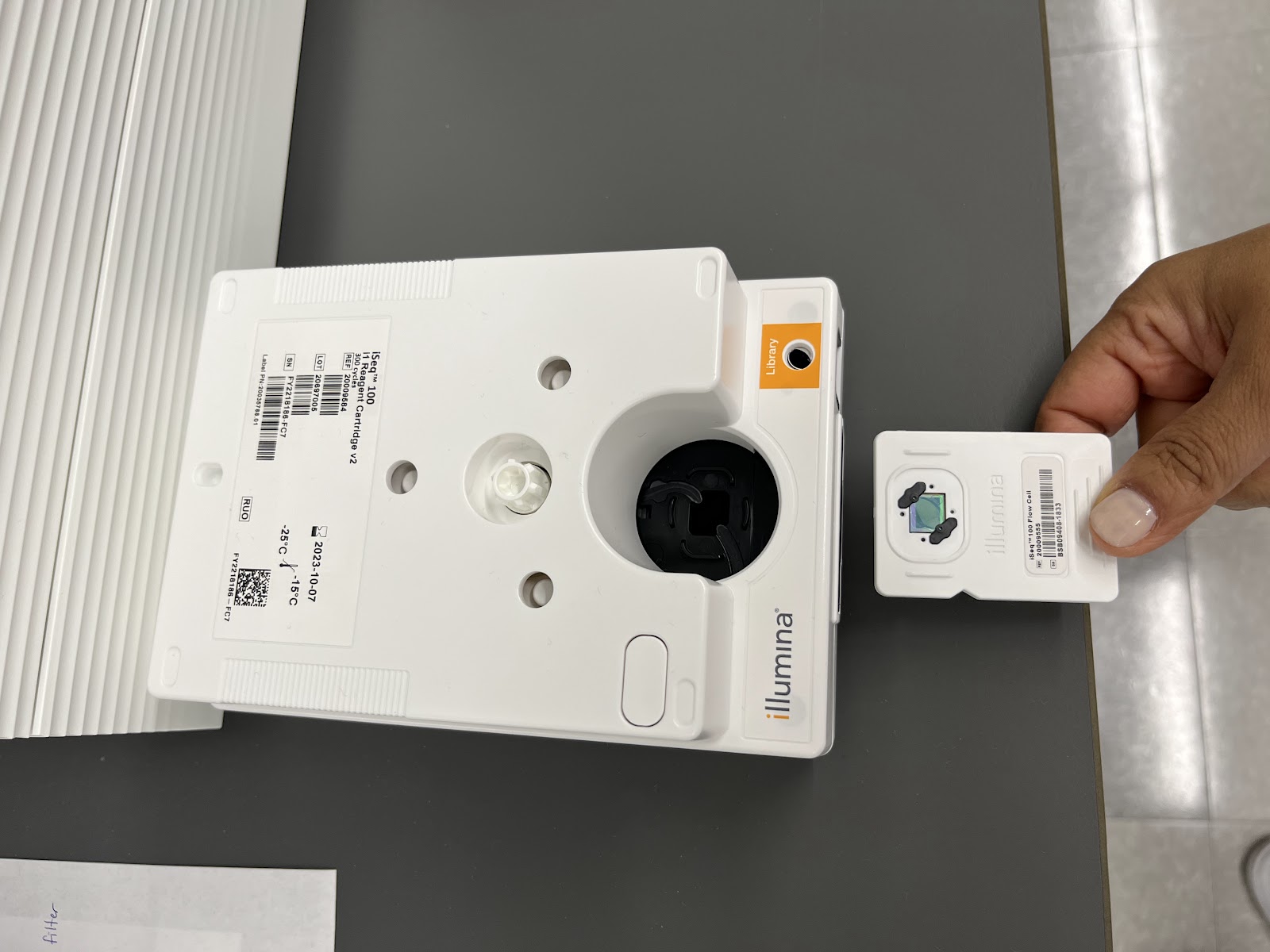
Above: Illumina iSeq 100, a smaller and more affordable model. Courtesy of Illumina.
Right: Illumina iSeq 100 flow cell, which researchers use for smaller genome sequencing because it is cheaper per sample. Many labs use it as a test method before sequencing in more expensive machines. Courtesy of Vinicius Fu.
Since the SGT is not as space-constrained as the ISS and is more focused on high throughput, the facility uses massive apparatuses to optimize workflow. Similar to MinION, ONT has also developed GridION, which can work with five flow cells at a time. The process of preparing the genome that occurs before sequencing is also automatized using a Biomek i7, a sort of “mechanical hand.” Instead of pipetting thousands of samples and amplifying them in a thermocycler, this robot does the repetitive job following the setup determined by lab needs.
“There are two parts to DNA/RNA sequencing. The first is that we have to prepare the samples in libraries, where we chop up the genome into little bits and put on specific adapters that can be put in the sequencers,” explains Dr. Lenz.
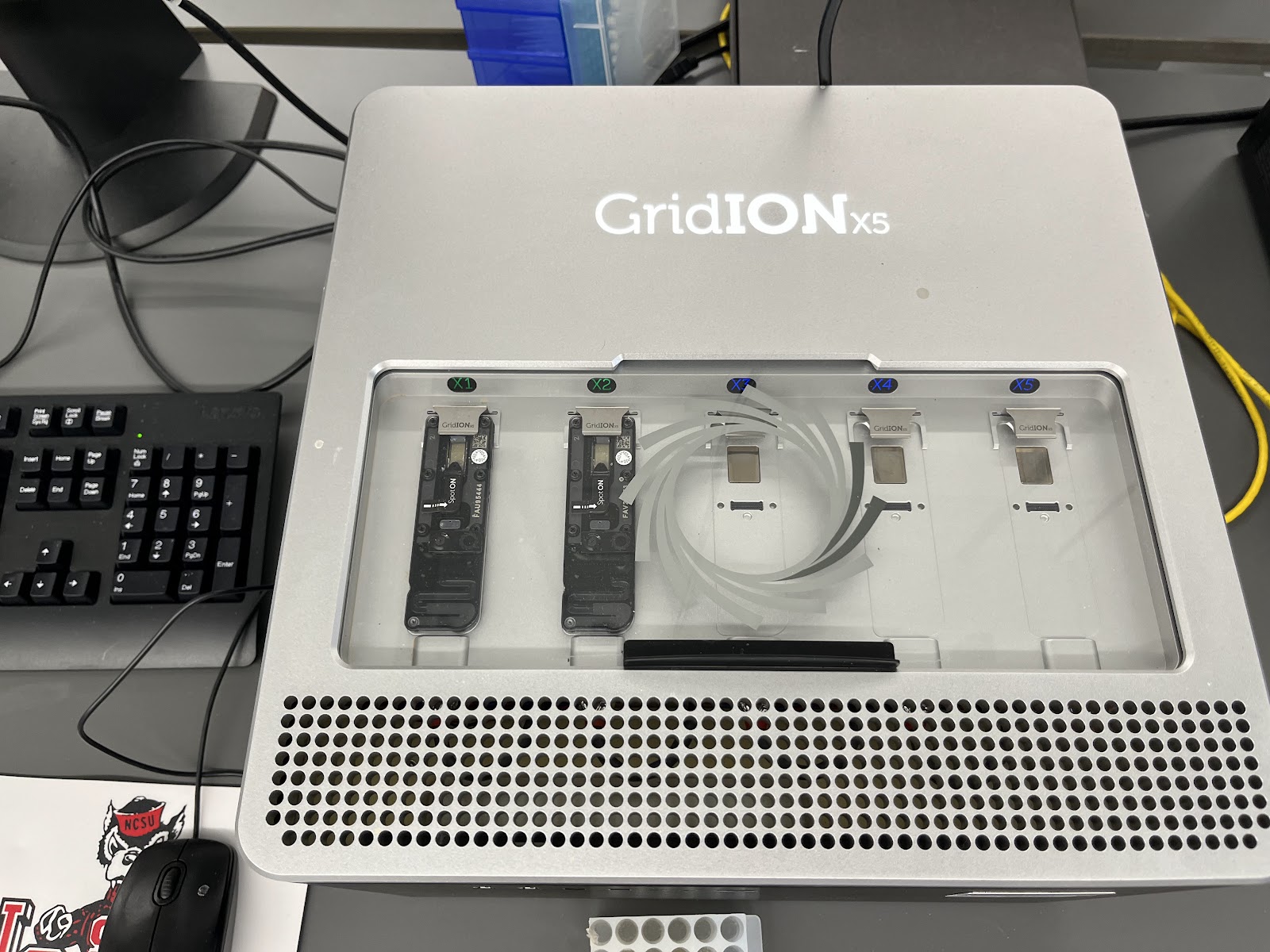
Above: GridION X5 by Oxford Nanopore Technologies. While it can fit up to five flow cells at a time, only two flow cells are present in this image. Courtesy of Vinicius Fu.
Right: Biomek i7 automated liquid handling workstation. It improves efficiency in labs that require high throughput. Functions include liquid mixing and PCR for library preparation before starting sequencing. Courtesy of Vinicius Fu.
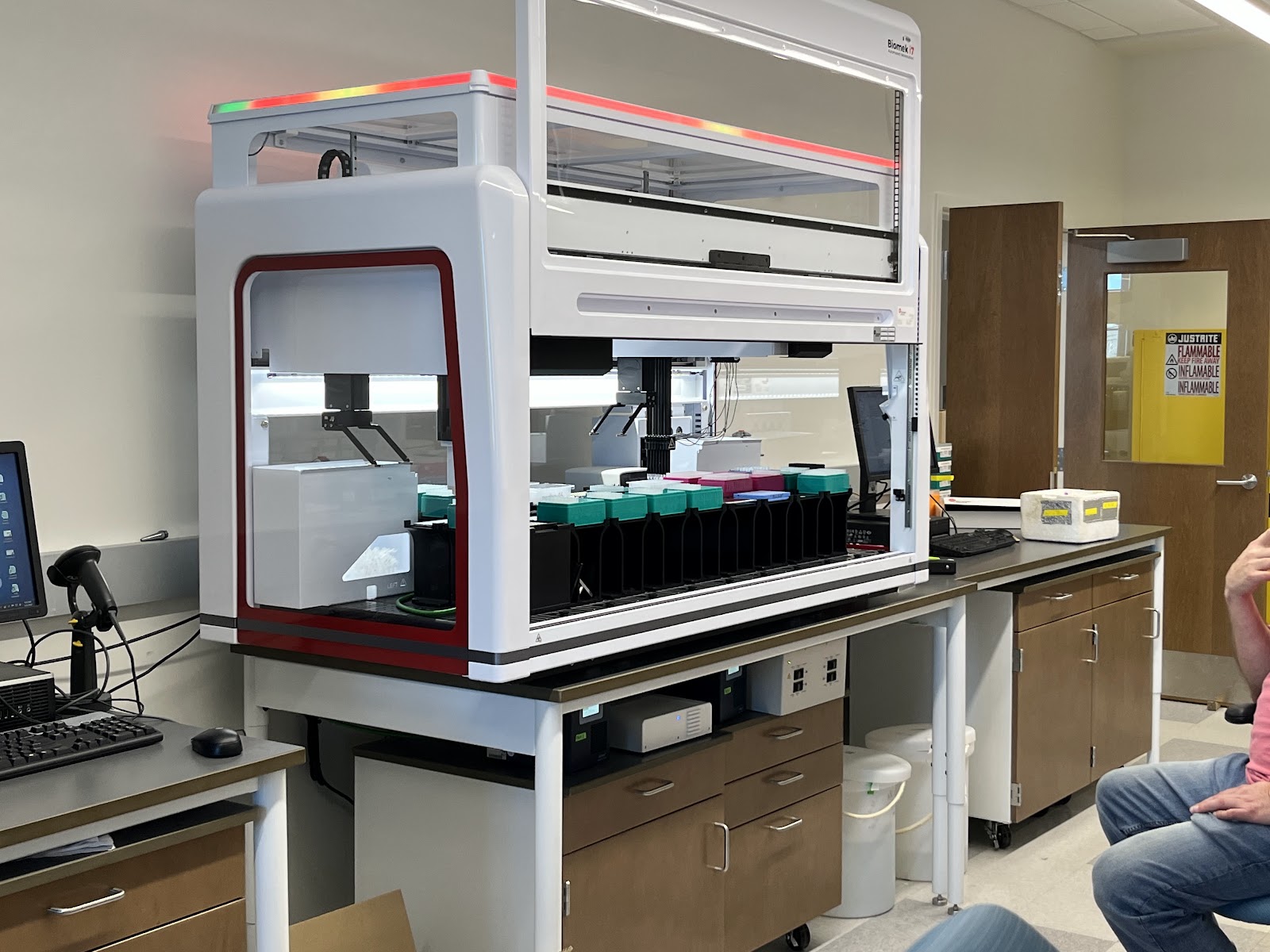
These novel technologies have helped astronauts control their mold problem. Indeed, the researchers found one major fungal culprit contaminating the ISS to be the common black mold Aspergillus niger. This particular fungus is highly resistant to space radiation and microgravity. Whether NASA will be able to permanently solve its mold issue remains uncertain; however, rapid biotechnological innovations are on the horizon to aid them in this expedition. Their success with genetic sequencing in space illustrates just how far scientific development has come and opens doors to uncovering the evolutionary secrets that have been concealed in genomes for billions of years.