The central dogma is a core concept of biology and genetics, but do these famous ideas still hold true today? Throughout the 20th and 21st centuries, scientists have added numerous amendments to this fundamental biological process, revolutionizing our understanding of how our genes shape development. This year’s Nobel Prize in Physiology or Medicine honored one of the most substantial contributions to our understanding of genetic information flow.
The Central Dogma
Since 1958, the central dogma has been cemented as a fundamental process in biology. It describes the transcription of DNA into mRNA and then translation into proteins. Moreover, numerous levels of regulation allow one genome to code different proteins needed by varying cell types despite possessing the same fundamental genetic code. This highly specified process allows the human genome to create over 400 different cells, driving the diversity of life.
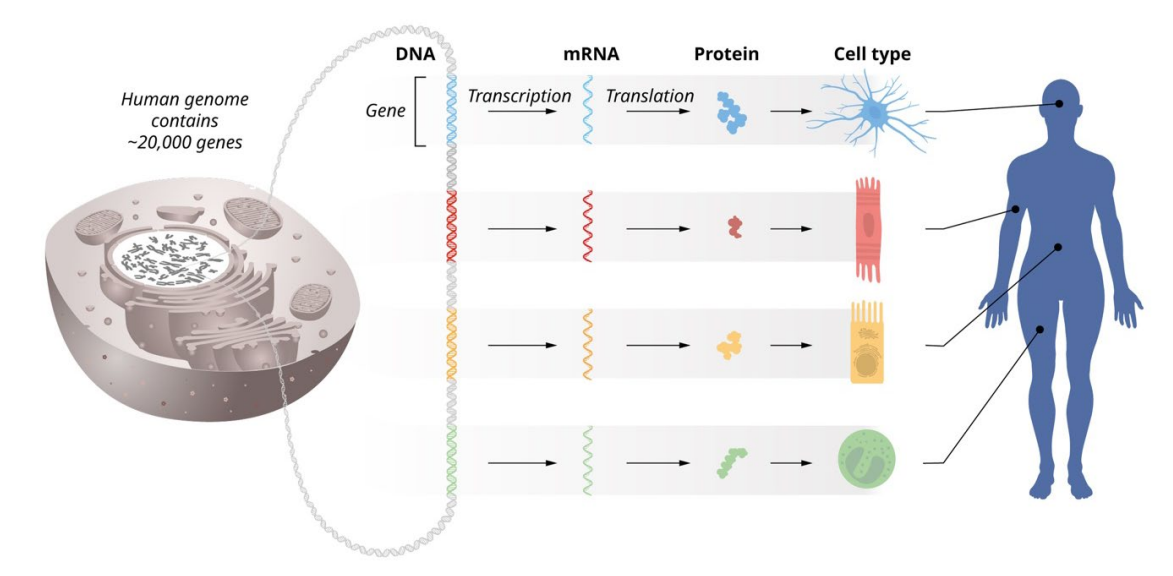
Above: Graphic of gene regulation specificity and the central dogma in different human tissues. Image courtesy of the Nobel Prize Foundation.
While scientists have previously discovered gene regulatory proteins, Victor Ambros and Gary Ruvkun’s discovery of microRNA (miRNA) introduces a new mechanism involved in post-transcriptional regulation of gene expression.
What is microRNA?
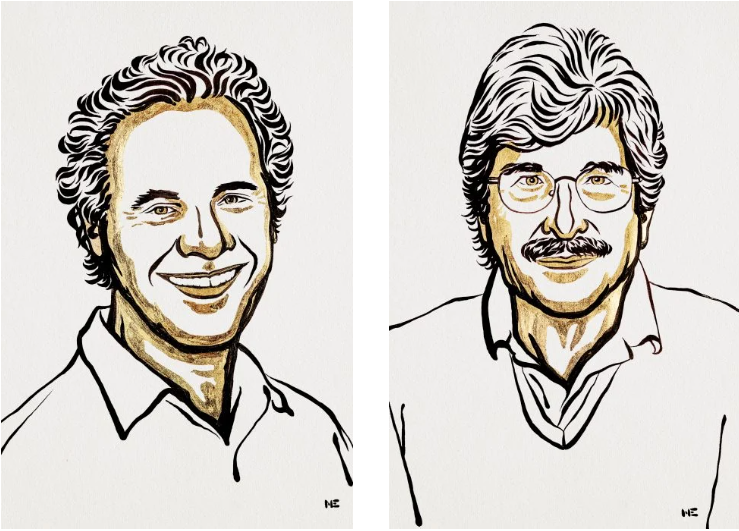
Above: Victor Ambros (left) and Gary Ruvkun (right), winners of the 2024 Nobel Prize in Physiology or Medicine. Image courtesy of the Nobel Prize Foundation.
In 1993, the two postdoctoral researchers had separately made novel discoveries about gene regulation in C. elegans, a transparent worm used as a model organism for research. Ambros and Ruvkun initially studied lin-4 and lin-14 mutations in C. elegans development. They each hypothesized that lin-4 encoded a repressor of lin-14 transcription, modulating the activation and deactivation of these genes and controlling the timing for the development of C. elegans.
At this time, Victor Ambros mapped the length of the lin-4 gene. He found that the transcribed RNA was shockingly short—simply 28 nucleotides. Ambros reasoned it would have been impossible for a sequence this short to code for a functional protein. Concurrently, Gary Ruvkun discovered that lin-14 mRNA is still present despite functional lin-4 genes. The combination of these findings allowed Ambros and Ruvkun to collaborate. More detailed mapping of the specific nucleotides in the mRNA revealed that the transcribed lin-4 gene matched an untranslated region of the lin-14 mRNA. Thus, Ambros and Ruvkun named the transcribed lin-4 a “microRNA” and suggested that miRNAs effectively block the translation of other mRNAs by binding the regions where translation would begin.
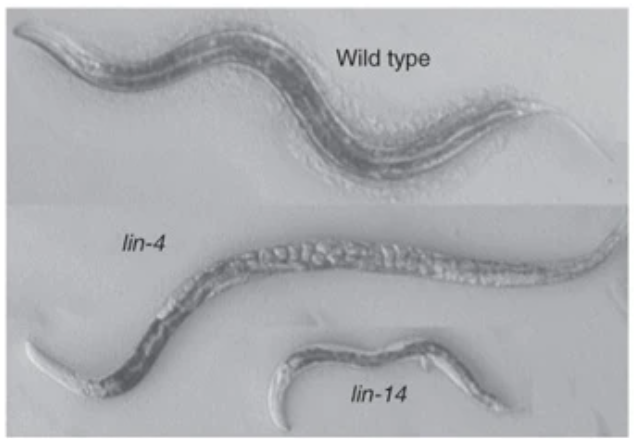
Above: C. elegans phenotypic change due to lin-4 or lin-14 mutations. Image courtesy of Ambros, 2008.
Although groundbreaking, the scientific community initially met these findings with skepticism. Because lin-4 and lin-14 are genes specific to C. elegans, many scientists believed miRNA was a phenomenon specific to these organisms. However, Ruvkun later discovered other highly conserved genes that also employed miRNA to regulate their expression. Ambros and Ruvkun found similar results using let-7, a gene encoding miRNA for most organisms. Because this gene is highly conserved, it was safe to assume that these organisms all used miRNA for gene regulation.
Furthermore, scientists Andrew Hamilton and David Baulcombe simultaneously discovered small interfering RNA (siRNA), which performs a similar function to miRNA. While miRNAs bind imprecisely and reversibly, siRNAs destroy their target using precise binding. The discovery of these small yet mighty RNAs greatly contributed to scientists' current understanding of post-transcriptional regulation.
Future Directions
This year, the Nobel Prize committee recognized the importance of this work in elucidating the roles of miRNA in genetic regulation and the applications of these discoveries in medicine. Throughout the decade, researchers have made many more exciting discoveries about the roles of various miRNAs and related complexes.
For instance, in addition to inhibitor miRNAs, researchers also discovered translation activator miRNAs. These nucleotides increase the rate of translation by guiding ribosomes to the translation initiation region. Furthermore, various miRNAs are localized in different organelles, allowing the specific miRNA to increase or decrease protein synthesis in a particular region and creating another layer of gene regulation.
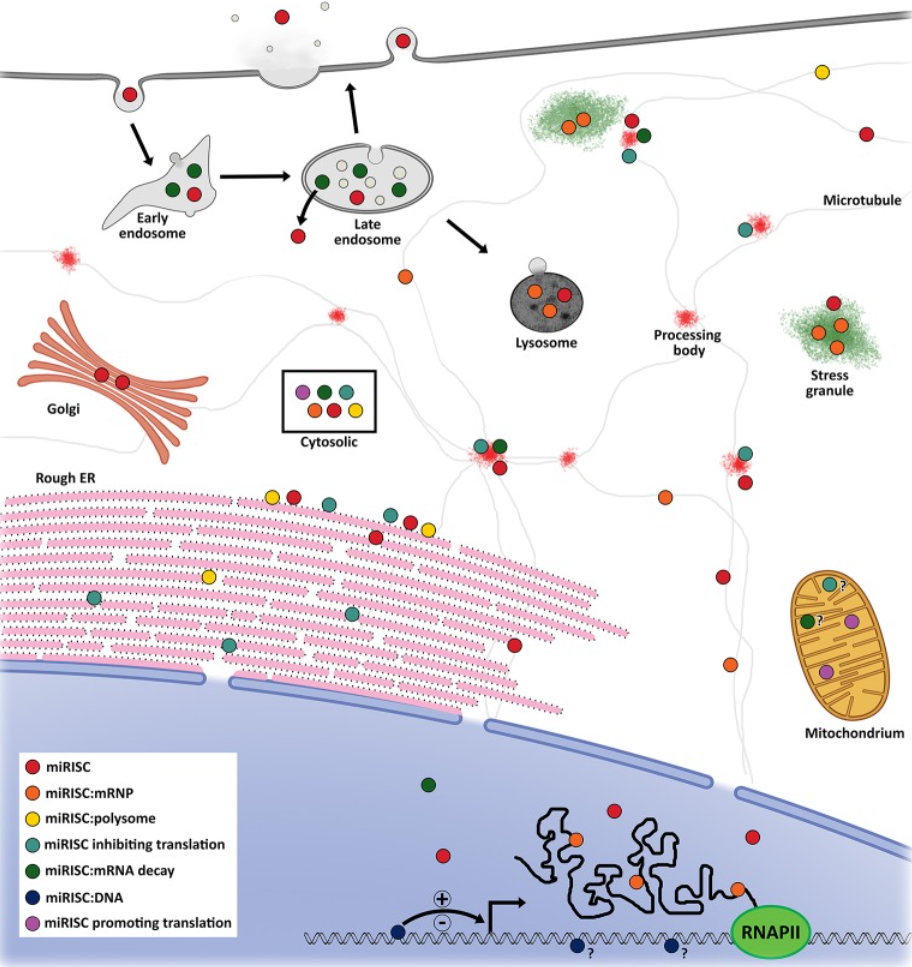
Above: Proposed model of the locations of various miRNAs. Image courtesy of O’Brien et al., 2018.
New research has sought to understand the importance of miRNA functions. While our understanding of miRNA has grown since the 1990s, scientists maintain a limited understanding of miRNA’s interaction with its surroundings. This research could lead to numerous therapeutic advances for various diseases by up-regulating or down-regulating protein expression. Many pharmaceutical companies have invested in this field and progressed miRNA therapies through the drug pipeline, including a hepatitis C treatment that underwent clinical trials. While the treatment has not proceeded to Phase 3 of clinical trials, researchers continue to study the mechanisms of this particular miRNA. Ultimately, these new efforts to advance our understanding of RNA in genetic regulation are both exciting and potentially revolutionary.
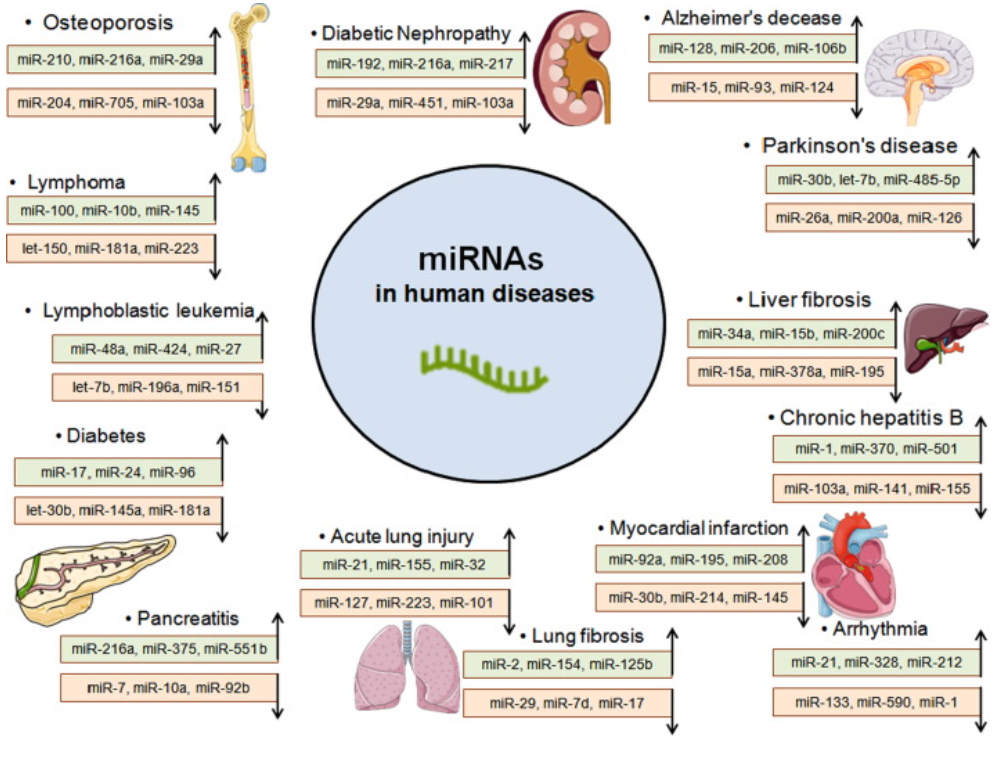
Above: Potential miRNAs that can be used to treat various human diseases. Image courtesy of Chakraborty et al., 2021.