A Persistent Homology Analysis: Hallucal Metatarsal Morphology and Primate Grasping Abilities
By Ksenia Sokolova, Yuqi Yun, published in our 2016-17 issue
Abstract
Persistent homology is a method in topological data analysis to extract topological features from data and measure the resolution of those features under different scales of observation. The morphology of Metatarsal 1 (Mt1) is argued to have a correlation to the grasping specializations of primates. Analyses of the characteristics of these bones such as peroneal process length, thickness, torsion and physiological abduction angle can be used to reconstruct the locomotion patterns for extant species.
We analyzed the dataset associated with different grasping types (non-grasping, rudimentary grasping, small-branch grasping, semi-terrestrial grasping, terrestrial grasping, vertical clinging and leaping, vertical grasping). For each species, a set of corresponding descriptive statistics and a surface file were assigned. Descriptive statistics included values for the peroneal process length, thickness, torsion and physiological abduction angle. We used persistent homology to analyze both descriptive statistics and surface files. Analysis of the descriptive statistics exhibited a clustering of non-grasping and rudimentary grasping separate from other types, but failed to distinguish between the types of grasping abilities. Principal component analysis showed that torsion and peroneal process length, normalized with respect to the geometric mean, accounted for most of the variation (99.99%) within the dataset. Persistent homology analysis of the bone surfaces using filtration by height in x-,y- and z- directions showed pairwise differences between some types of graspers (such as terrestrial and vertical graspers), though there was no evident clustering within the persistence diagrams for all types of graspers.
Introduction
Morphology of Metatarsal 1 and Grasping Ability
Morphology of the hallucal metatarsal can be used to reconstruct the grasping ability and locomotion mode for the fossil taxa and to analyze the evolutionary history of the locomotion. Metatarsal 1 plays an important role in the propulsive movements for arthropods (non-graspers). At the same time, it facilitates grasping for other species. Therefore, the morphological features of Mt1 are significant and provide insight into functionality and locomotion.
Data Sets
Two distinct data sets were used. The first data set contained the descriptive statistics for hallucal metatarsals taken from the paper by Goodenberger et. al. In this paper, authors analyzed the relationship between the morphological characteristics of Mt1 as well as their relation to grasping ability and the evolution of pedal grasping. Features believed to be related to grasping ability were measured for each specimen: torsion, peroneal process length (PPL), peroneal process thickness (PPT) and physiological abduction angle (PAA). Peroneal process length and thickness data were normalized both by the geometric mean (GM) and the cubic root of body mass (BM). Therefore, each metatarsal was associated with six descriptive values and, according to the grasping ability of the species, was accordingly catalogued into non-grasping, terrestrial grasping, semi-terrestrial grasping, large branch grasping, small branch grasping and vertical grasping categories.
We obtained the second data set from Morphosource.org, an open access web-archive that allows users to access 3D surface scans of bones including fossil samples. The scans represent the meshed surface of the bone, obtained by a micro-CT scanner. X-ray projections of the bones are taken from multiple directions and converted into a stack of images representing the cross-sections of the bones. Each stack is then used to create a 3D-surface. This is a direct 3D imaging method, which generates a surface directly based off of the imaging measurement. The resulting surface files allow for us to analyze the morphology of the bones, without relying on the individual measurements between the surfaces which are prone to human error and might not provide the full information about the structure.
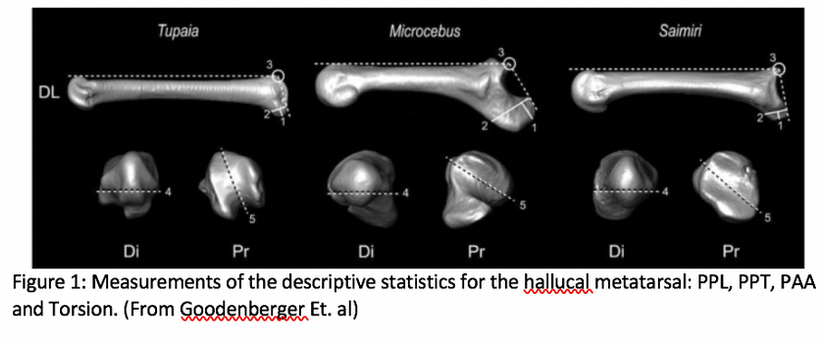
Expected Results
First, when analyzing the descriptive statistics, we wanted to see clustering based on grasping abilities. We also wanted to find which values among the descriptive statistics explained the most variation within the set, using persistent homology to assess potential clustering. We anticipated that using Principle Component Analysis (PCA) to determine the most significant data points would allow us to visualize the data.
When analyzing the surface files we used persistent homology on the set to attempt to find the global features of the high dimensional data that may not have been available by straightforward measuring. We hoped to find a method of analyzing the surface of the bone so that the 0-dim or 1-dim persistence diagram would exhibit clustering. This would allow us to classify the bones based only off of the surface files.
Persistent Homology of Descriptive Statistics
We constructed a six-dimensional point cloud in which each point represents the measurements of the six descriptive statistics (torsion, PPL/GM, PPL/BM, PPT/GM, PPT/GM, PAA, Figure 1) of an individual species among 81 representative species. When performing a filtration of the Vietoris-Rips complex, the zero-dimensional analysis gives the degree of connectedness of the data in the point cloud. In this process, the points in the point cloud may form persistent clusters which usually have important physical meanings.
The six descriptive statistics (torsion, PPL/GM, PPL/BM, PPT/GM, PPT/GM, PAA, Figure 1) of each specimen are converted to a point in six-dimension. We constructed a point cloud with 81 representative primate species and analyzed the resulting Rips complex using Topological data analysis (TDA). First, we intended to study whether species with the same or similar grasping behaviors would form a cluster. The one-dimensional persistence diagram of the Rips complex showed three points with relative high persistence, which indicated the formation of two to four clusters based on our tolerance (Figure 2). However, a dendrogram that visualized this hierarchical clustering process showed that only the highest persistence is meaningful in terms of grasping ability (Figure 3). The non-grasping and rudimentary grasping species formed a group that was relatively distant from all other species, but the hierarchical clustering for grasping species appeared to be random. This result mainly agreed with the results from the box-and-whiskers plots in Goodenberger et. al., which show the distance between graspers and non-graspers but not among different types of graspers.
Principal Component Analysis of Descriptive Statistics
Principal component analysis (PCA) shows that among the six descriptive statistics, the most pivotal ones are torsion and PPL/GM. The two features explained 77.3796% and 22.6115% of the data, respectively. A two-dimensional point cloud with only torsion and PPL/GM was then constructed, and we repeated the persistent homology analysis of the resulting Rips complex (Figures 4, 5). The zero-dimensional analysis yielded two points with high persistence, similar to the previous result. Thus, PCA should mostly preserve the previous clustering. By reducing the dimension of the data, we were able to visualize the point cloud as a scatter plot in two-dimension, where non-grasping and rudimentary grasping species appeared as outliers, consistent with the previous result.

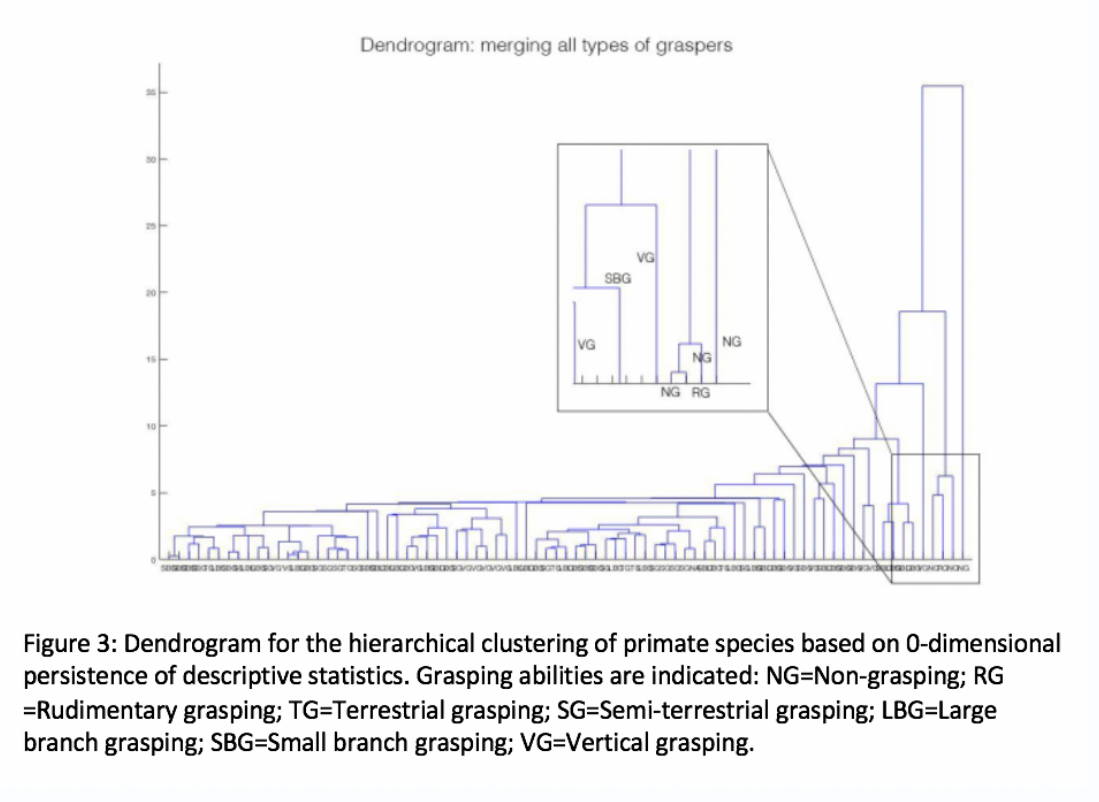
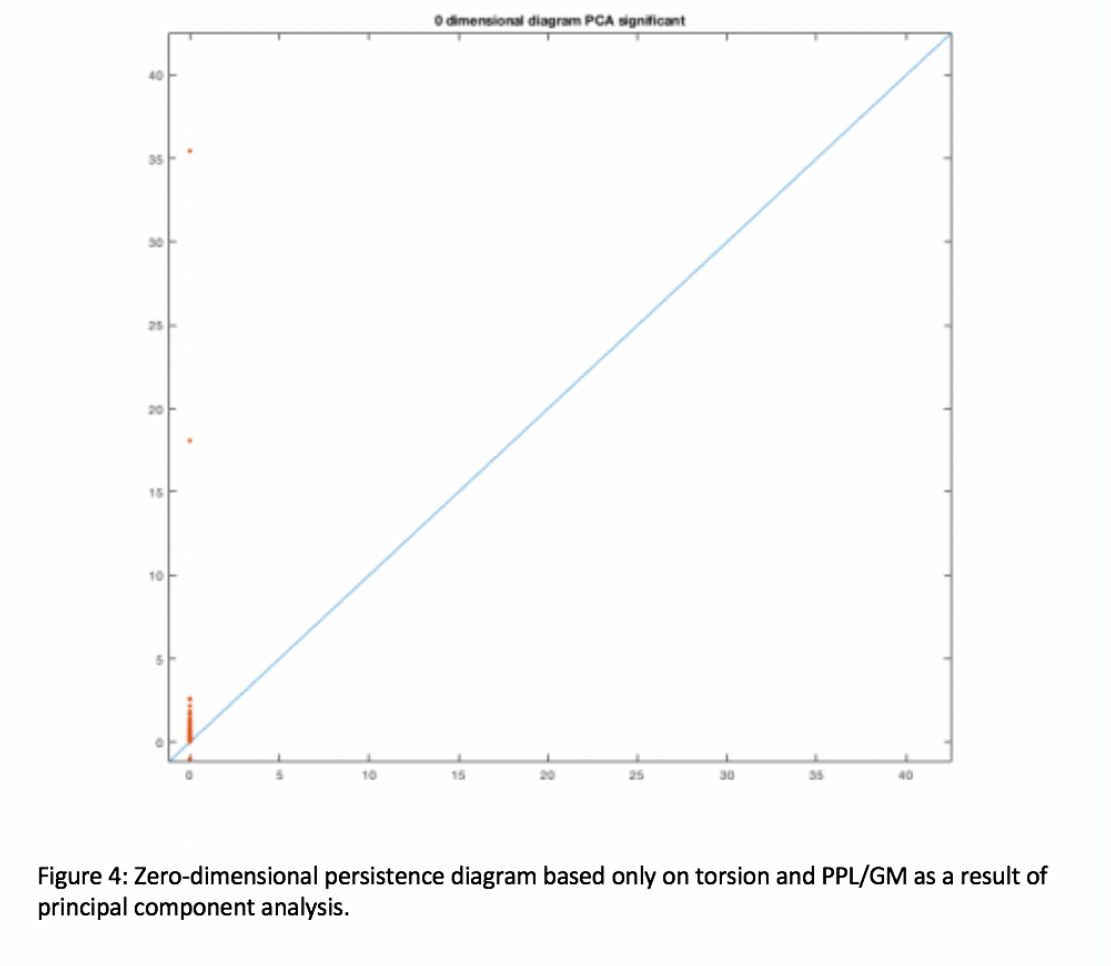
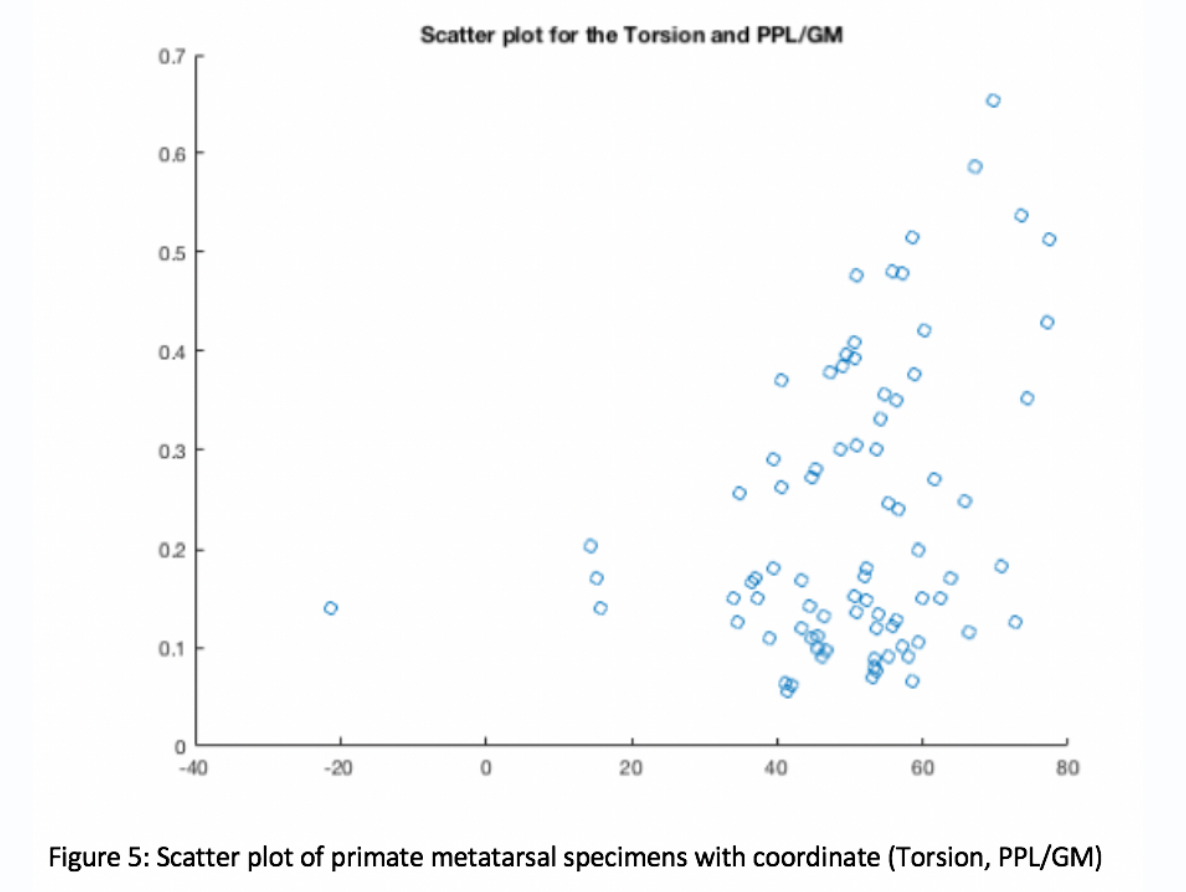
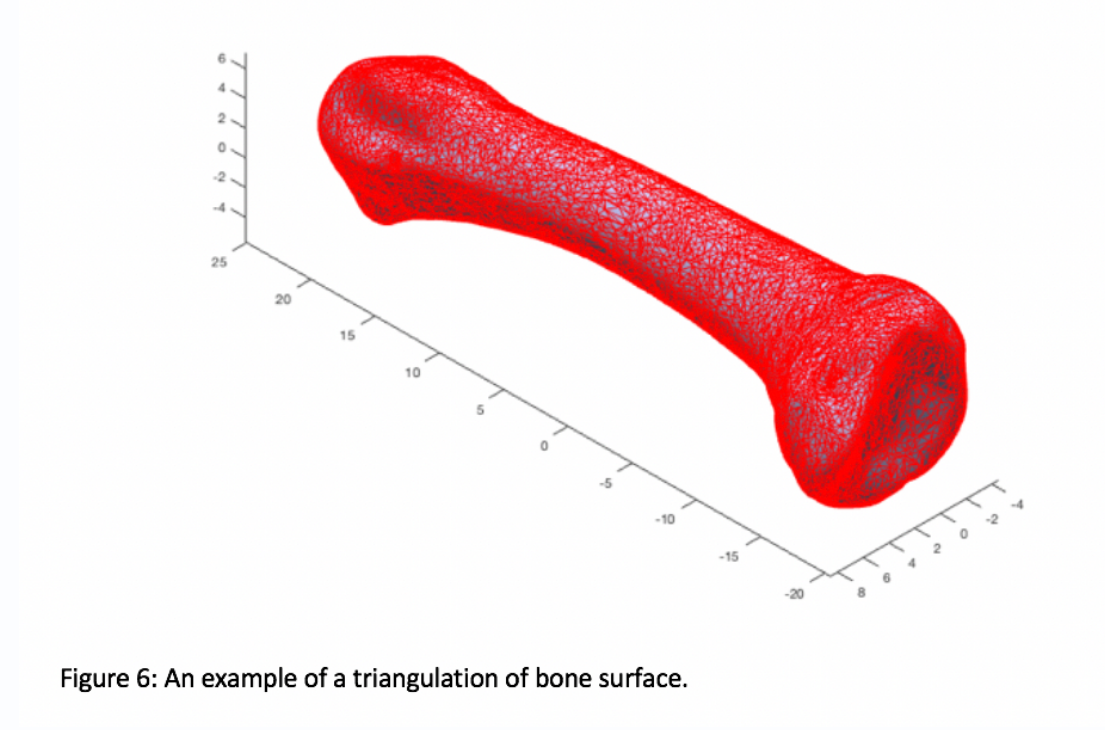
Persistent Homology of Surface Scans
Triangulation and Alignment of Bone Surface Scans
In the conventional study of descriptive statistics, only limited features of the bone are used for analysis. These are often difficult to measure and they are subject to human error. Topology data analysis (TDA) offers a way to minimize pre-processing and to study the bone surface as a whole. To apply TDA, we triangulated each bone surface by converting the CT scan into a mesh in Matlab (Figure 6). The alignment was done through centering, scaling and reorienting the bones. We centered the bones by choosing the minimum coordinate value in the direction we filtered to be zero. It ensured that the first component in zero-dimensional persistence analysis was born at time zero. All bones were scaled to be approximately the same length as a reference bone. Bone samples with apparent misaligned orientation were excluded from the following analysis.
Filtration of Bone Surface by Height Functions
The information about the triangulation was stored in an N-by-3 matrix representing N number of faces, each with three vertices. We defined the height function assigned to each vertex to be the Cartesian coordinate values of the vertex in the direction of filtration. The height function assigned to each edge was then the larger height value of the two vertices on the edge’s boundary. Using these assignments, we studied the zero- and one- dimensional persistence for each metatarsal specimen with height filtrations in x-, y- and z- directions. Among the height filtrations, only the filtration with respect to the z-direction yielded interesting features.
We superimposed zero-dimensional persistence for four Homo Sapiens metatarsals (Figure 7) to calibrate our method. Similarity between the diagrams showed that the persistent homology of the same species are close to each other, exhibiting little variation.
We then compared the features of persistence among different grasping types. The zero- (or one-) dimensional persistence diagrams of species in the same grasping category were merged to represent the persistence feature of that category overall. By comparing the merged one-dimensional persistence diagram between terrestrial graspers and vertical graspers, we observed that they formed two separate clusters. This result, which was unobtainable with descriptive statistics, showed that the differences in metatarsal morphology between the two types of graspers may count for the difference in grasping abilities, or vice versa. This pairwise difference could also be found through comparing limited numbers of other combinations such as non-graspers and vertical graspers (Figure 8).
Although the above result appeared promising, the height filtration in the z-direction overall was not sufficient to distinguish among all different types of graspers. When we compared the merged persistence diagrams across all grasping categories, the persistent points for both zero-dimension and one-dimension were almost perfectly mixed together -- no distinguishable cluster for any single grasping category existed (Figures 9, 10).
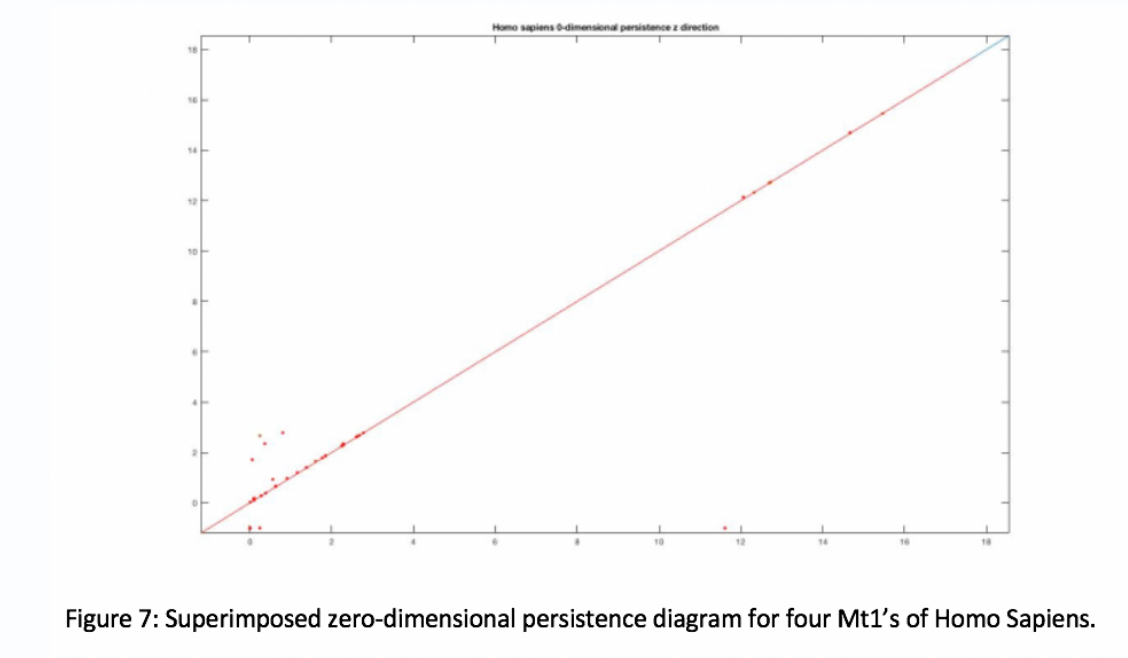

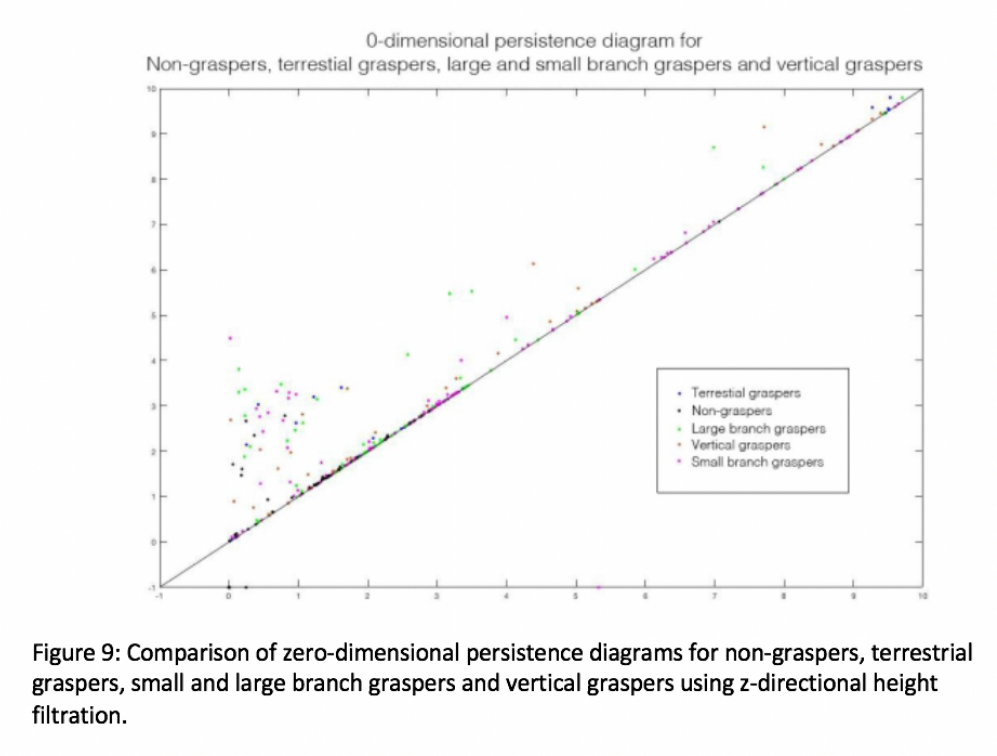
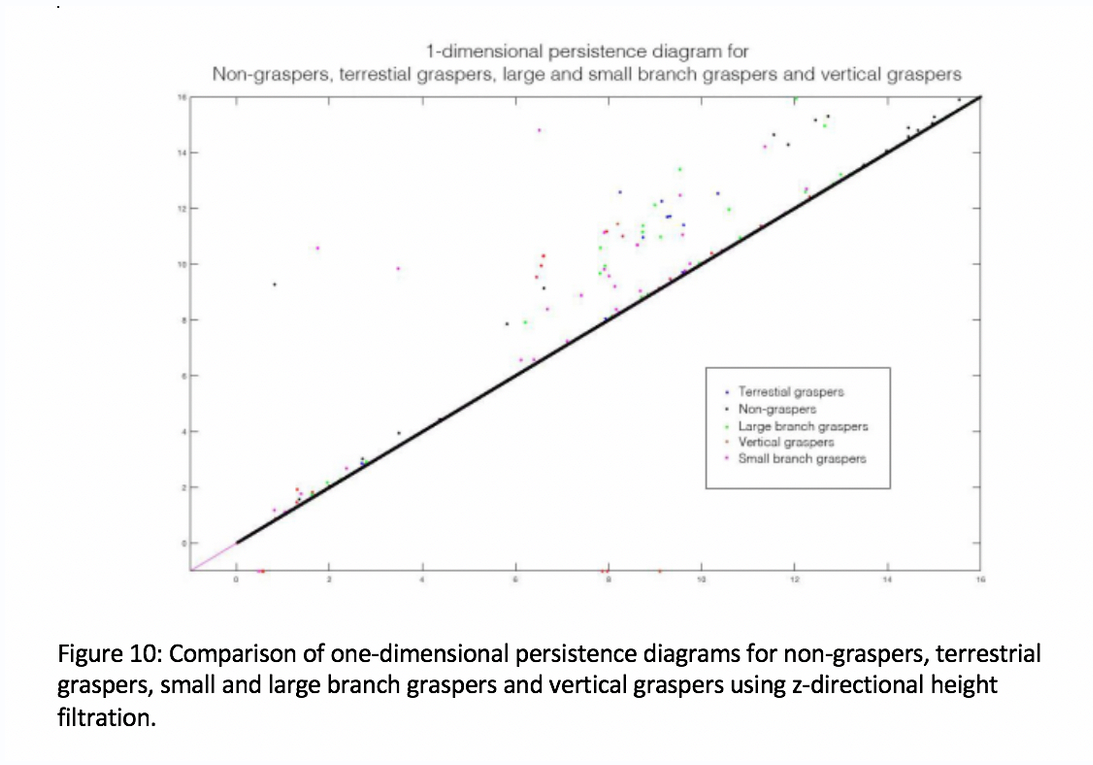
Discussion
We can see from the analysis of the first data set that the descriptive statistics are limited to distinguishing between grasping and non-grasping species only. It is possible that either the descriptive features we had chosen were not sufficient in categorizing the differences between metatarsals, or that the metatarsals themselves do not differ significantly according to grasping ability. We have also seen that almost all of the variation can be explained by torsion and PPL/GM. This observation is consistent with the statistical results [1] of analyzing the data set.
Analysis of the surface files showed no points with high persistence in x and y directions. However the z-direction showed some interesting clustering. The one-dimensional diagram in the z- direction allowed us to distinguish between terrestrial graspers and vertical graspers, and there was observable clustering in the one-dimensional diagram for non-graspers and vertical graspers. However, when we overlaid the persistence diagrams of all types of graspers we saw zero clustering in either dimension. This may be due to variation within the original positioning of the metatarsal, scaling or a lack of observable features.
In future work, the most important improvement to this method would be to align and normalize the bones using anthropologically justified methods. Another possible option would be to run persistence from different angles beyond the three directions (x,y,z) and then analyze the resulting diagrams. It would be also possible to use PCA to find the average bone within the grasping category and analyze variations within these bones. This would signal whether there is excessive intra-species variation.
Conclusion
Overall, this study showed that some morphological features of the hallucal metatarsal (both descriptive statistics such as torsion and the length of the peroneal process as well as bone surface shape) may to some extent serve as a measure for distinguishing taxa belonging to different grasping groups. However, these methods can only be applied to certain combinations of grasping categories and are of limited accuracy. Therefore, these morphological features alone cannot enable us to reconstruct the grasping ability of extant primates, though they may be used in supplemental methods for providing new insights on behavioral evolution throughout primate lineage.
Works Cited:
K. Turner, S. Mukherjee, D. M. Boyer. Persistent Homology Transform for Modeling Shapes and Surfaces. arXiv: 1310.1030v2. 15 June 2014.
K. E. Goodenberger, D. M. Boyer, C. M. Orr, R. L. Jacobs, J. C. Femiani, and B. A. Patel, Functional Morphology of the Hallucal Metatarsal With Implications for Inferring Grasping Ability in Extinct Primates. American Journal of Physical Anthropology 156:327?348. 2015
N. Part, J. Lee, K.H. Sung, M.S. Park, and S.Koo. Design and Validation of Automated Femoral Bone Morphology Measurements in Cerebral Palsy. Journal of Digital Imaging J Digit Imaging 27.2: 262-69. 2013